Neuroscience Breakthroughs Since Graduate School - Part 2: Addiction
- BioSource Faculty
- Nov 7, 2024
- 17 min read
Updated: Feb 20

Addiction inevitably involves loss, including executive functions, health, pleasure from everyday life, productivity, and often relationships.
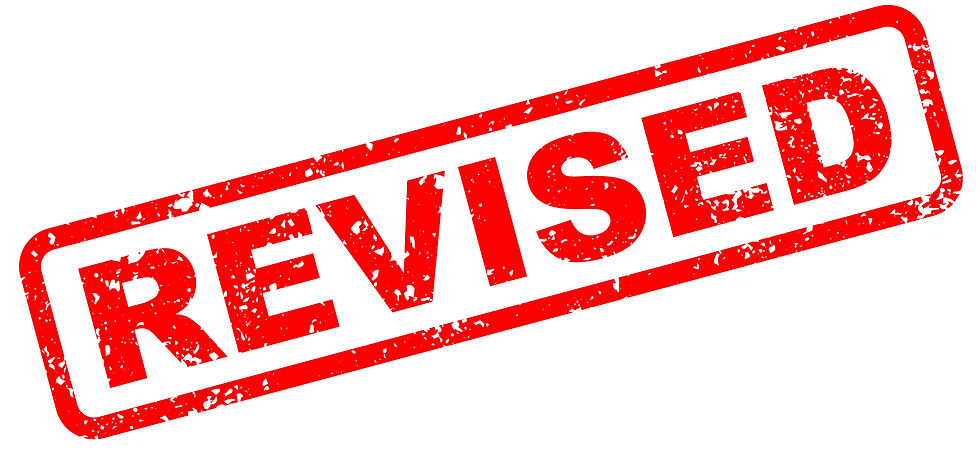
There has been a paradigm shift to a more complete model of addiction that combines negative and positive reinforcement. Addiction impairs an individual's capacity to prioritize behaviors yielding long-term benefits over immediate rewards, and the growing challenge in controlling these behaviors, even when they carry severe consequences (Volkow & Boyle, 2018).
Although many factors contribute to substance use disorders, stress and deficient dopamine receptors may be crucial. Abused drugs cause direct and indirect dopamine release at the nucleus accumbens. While dopamine begins the reward cascade, addiction involves diverse neurotransmitters. Craving is one of the most dangerous threats to sobriety. Craving emerges from a complex network of subcortical and cortical projections. The loss of brain volume due to apoptosis contributes to hypofrontality, impairing an addict's ability to resist craving and causing relapse. Neurofeedback is an evidence-based treatment for substance use disorder. The Scott-Kaiser modification of the Peniston Protocol plus rehabilitation for substance use disorder has achieved a rating of probably efficacious, comparable to accepted medical treatments. This second installment focuses on the neuroscience of addiction and the efficacy of neurofeedback interventions.
Alcohol is cunning, baffling, and powerful (The Big Book of Alcoholics Anonymous).
A Paradigm Shift
The view before 1969 was that addiction is fueled by drug dependence. This negative reinforcement model proposed that addicts ingest their next dose to avoid or escape unpleasant withdrawal effects.
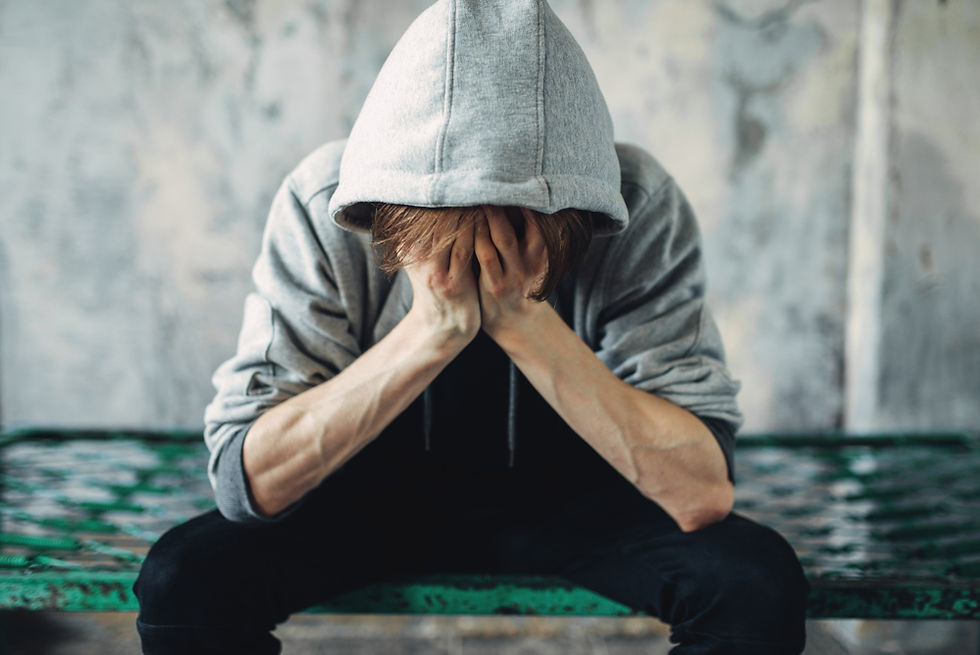
Negative reinforcement strengthens the behavior it follows. For example, an addict's "coke runs" can increase the likelihood of drug use by forestalling a cocaine "crash." This is different from punishment, which weakens the behavior by following it with an aversive consequence. Graphic © Suzanne Tucker/Shutterstock.com.
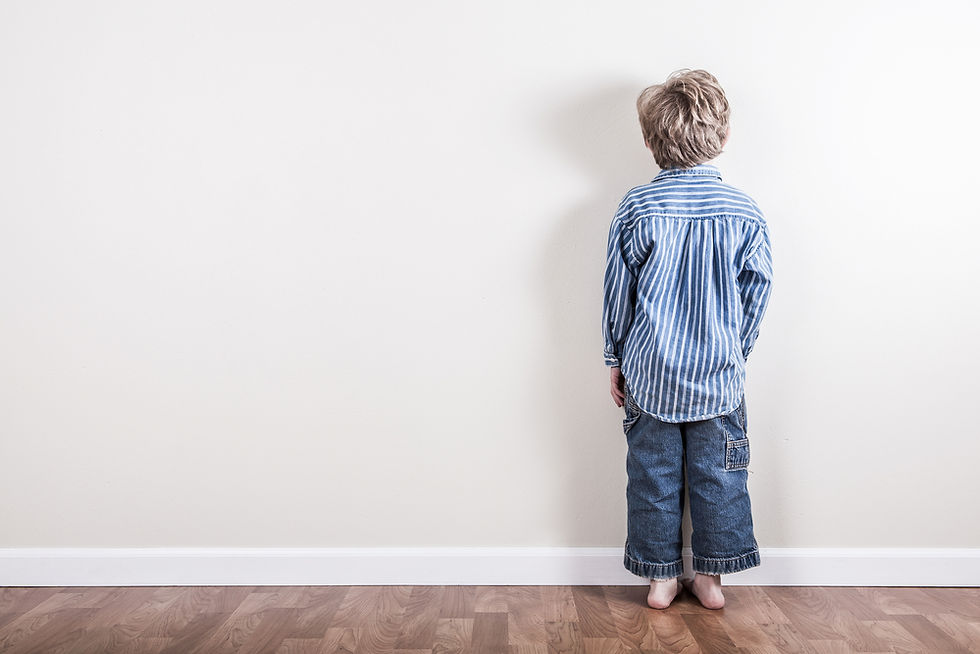
A tragedy of addiction is that punishing outcomes like arrests, job loss, and overdoses often cannot overcome drug craving.
The negative reinforcement model was incomplete because addicts crave drugs after completely withdrawing from them. Graphic © Jan H Andersen/Shutterstock.com.
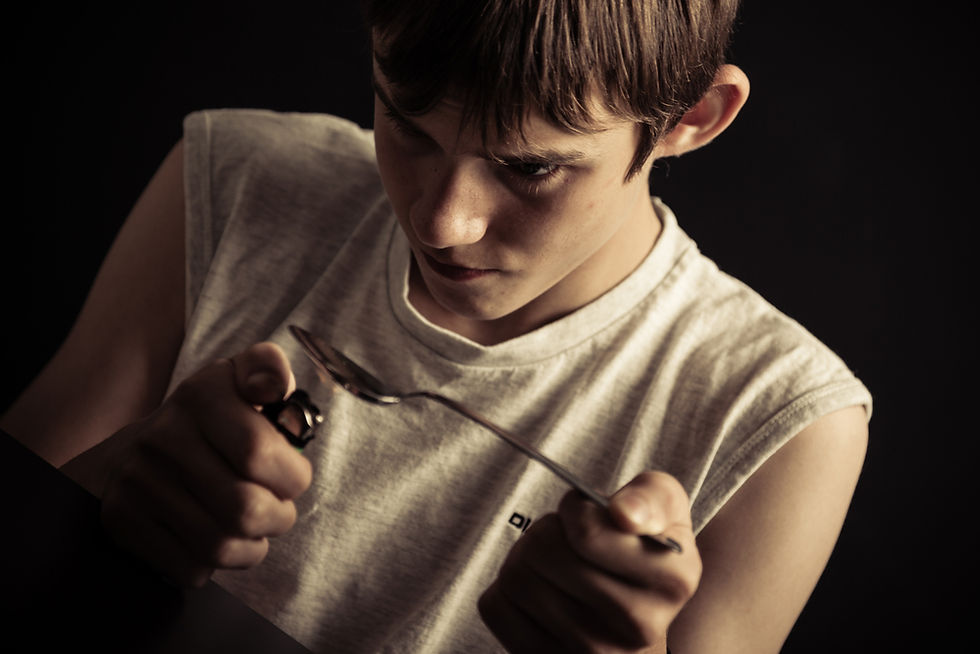
For example, Vietnam war veterans who had become addicted to high-purity heroin during their service suddenly relapsed after years of sobriety while back in the US. Graphic © Combatcamerauk/Shutterstock.com.
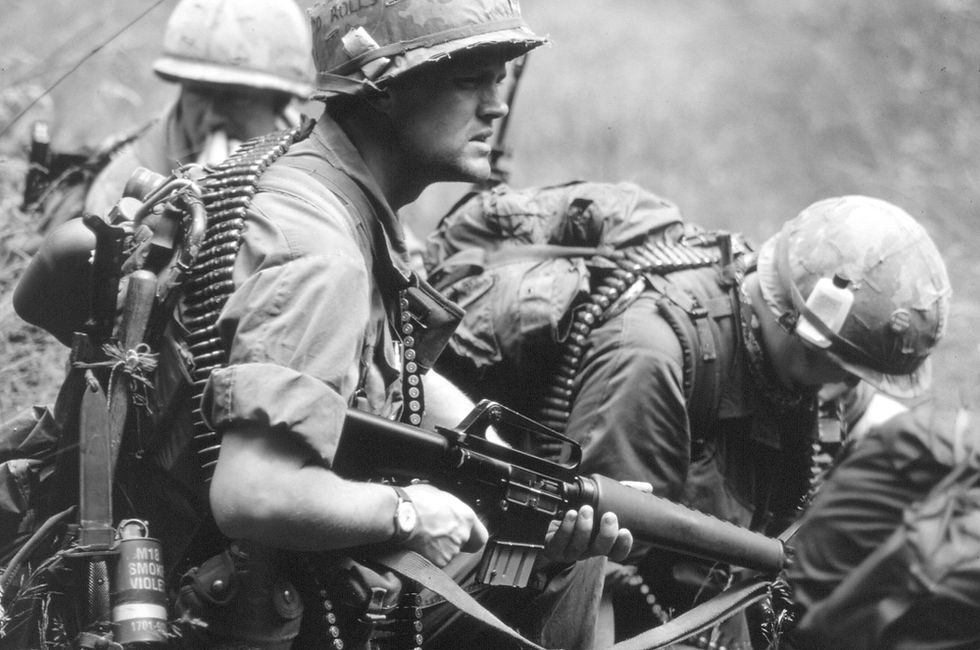
A more complete model of addiction emerged when researchers demonstrated that nonhuman animals will self-administer virtually all the addictive drugs abused by humans. Graphic © Jagodka/Shutterstock.com.
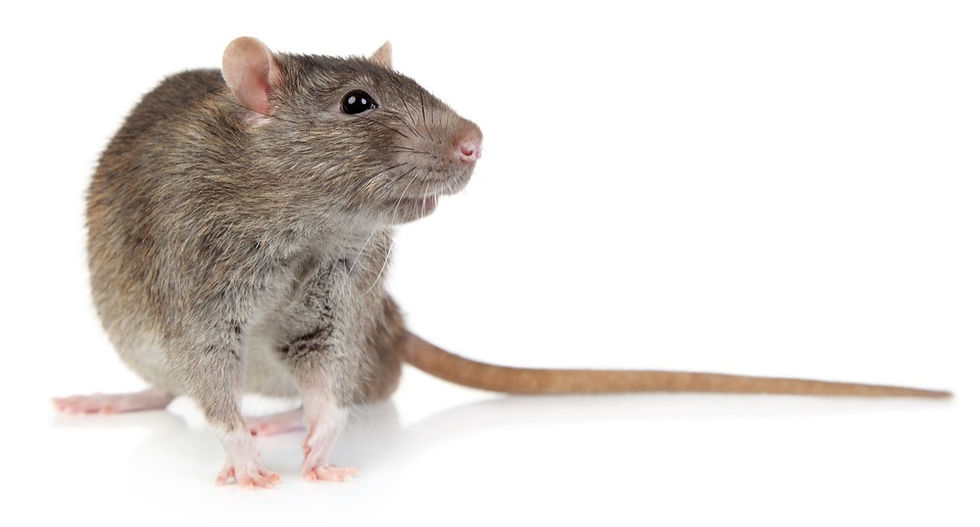
The revolutionary new view was that a drug’s reinforcing properties are due to its actions at specialized receptors. If you breed animals without the necessary receptor, they won’t self-administer the drug.
These studies showed that animals will:
1. inject abused drugs without physical dependence
2. prefer the place where they received the drug
3. expend considerable effort to obtain more of the drug
4. still work for the drug if it produces aversive consequences like shock
5. relapse after withdrawal when exposed to stressors, drug cues, and the original drug
Graphic © Evdokimov Maxim/Shutterstock.com.

Which other species does this resemble? Graphic © s_bukley/Shutterstock.com.
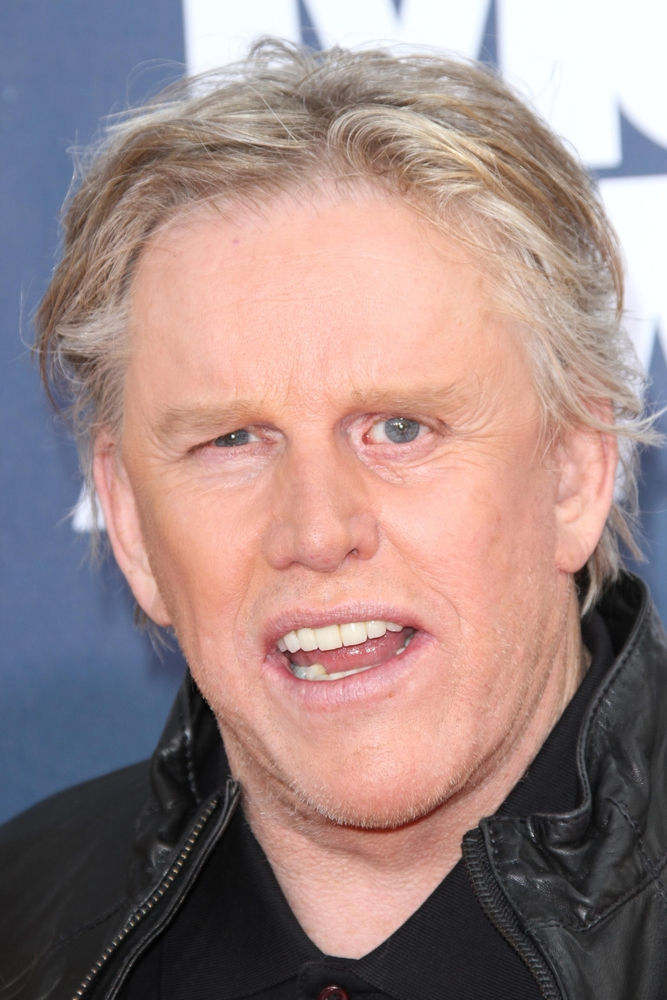
The Circuitry of Addiction
Dopamine encodes information about stimulus salience (relevance), including rewards and threats. The prefrontal cortex (PFC) evaluates craving-related information in response to drug-related cues. Networked structures include the orbitofrontal cortex (OFC), anterior cingulate cortex (ACC), and nucleus accumbens (NAC).
The hippocampus (HPC), insula, central nucleus of the amygdala, and the bed nucleus of the stria terminalis (BNST) consider affective states, context, and stress when processing craving-related information.
The Nucleus Accumbens
Drugs like cocaine and morphine interact with brain circuits that naturally process needs like hunger and thirst, thereby redirecting motivation to prioritize drug-seeking behaviors over essential physiological needs. Using advanced imaging and molecular techniques, Tan and colleagues (2024) identified the nucleus accumbens (NAc) as a critical area that integrates responses to natural and drug rewards. They found that drugs activate overlapping neuron groups with natural rewards but more intensely and disruptively, leading to enhanced activation with repeated use.
The researchers showed that both drugs engage two types of NAc neurons—those expressing D1 and D2 dopamine receptors. Cocaine primarily activates D1 neurons, whereas morphine stimulates D1 and D2 neurons. Over time, cocaine increasingly amplifies D1 neuron activity, while morphine amplifies both types, suggesting that these drugs manipulate the brain’s reward processing differently but within the same neural circuitry. During drug withdrawal, these patterns reverse, with cocaine withdrawal causing decreased D1 activity (reducing the response to natural rewards) and morphine withdrawal increasing D2 activity, often linked to negative affect, which may explain withdrawal’s aversive effects.
A critical molecular finding is the role of the protein Rheb in the NAc. Rheb, part of the mTOR signaling pathway, seems essential for drugs to override natural reward processing, as knocking out Rheb prevents drugs from disrupting feeding and drinking behaviors. This indicates that drugs exploit Rheb to remodel neural pathways selectively, adapting brain function toward prioritizing drugs over physiological needs.
Craving
Dorsolateral prefrontal cortex (DLPFC) activation increases craving by amplifying the response to drug-related cues through its connection with the OFC, ACC, and NAC. The ventromedial prefrontal cortex (vmPFC) exercises emotional control and inhibits actions. Conditioning and the loss of brain volume interfere progressively cripple these functions.
Craving is what makes addiction to drugs so difficult to overcome. The intense craving that follows a cue that has been previously associated with the drug, combined with a stressful state or a dysphoric state, represents an unstoppable force that leads to drug intake and relapse for most addicted individuals (Koob, 2013).
The Helplessness of Craving
A description of the physiological mechanisms mediating craving cannot convey a drug user's experience of profound helplessness. Their loss of control is intense and pervasive. When they relapse, addicts feel like a stranger in their brain is making disastrous decisions they cannot prevent. They feel helpless, terrified, hopeless, and ashamed.
A Two-Stage Cue Reactivity Model
Hayashi et al. (2013) proposed a two-stage model of cue reactivity. The medial orbitofrontal cortex (mOFC) determines a drug's subjective value based on self-reported craving. The DLPFC combines drug availability and cues in the context of time to adjust the mOFC estimation of drug value.
Multiple structures are responsible for craving, the intense motivation to acquire and ingest a drug. The dorsolateral prefrontal cortex (DLPFC) anticipates the effects of drug ingestion and estimates drug availability in the individual's situation. The DLPFC communicates its conclusions to the anterior cingulate cortex, orbitofrontal cortex, and nucleus accumbens. The anterior cingulate cortex (ACC) determines stimulus value by comparing the value and cost of acting on the stimulus. The orbitofrontal cortex (OFC) evaluates the drug's subjective value. Finally, the nucleus accumbens (NAC) promotes action in response to craving, communicating with the globus pallidus (GP) of the basal ganglia and the thalamus (Thal).
The vmPFC can inhibit action and regulate emotion through its connections with the NAC and central nucleus of the amygdala (CeA), which mediates emotional states. Action inhibition and emotional control progressively weaken as experimental drug use transitions to substance use disorder.
Neurotransmitters Involved in Addiction
Although many factors contribute to developing substance use disorders, stress and deficient dopamine receptors (e.g., low density or hypofunctional) may be crucial.
Stress
Stress contributes to developing an addiction and relapse (Haass-Koffler & Bartlett, 2012). Stress can precipitate mood disorders like depression in vulnerable individuals directly and indirectly through insomnia. Clinicians must treat two disorders when patients abuse drugs to manage their symptoms. Stress from everyday life and drug withdrawal can trigger drug craving and relapse. Graphic © El Nariz/Shutterstock.com.
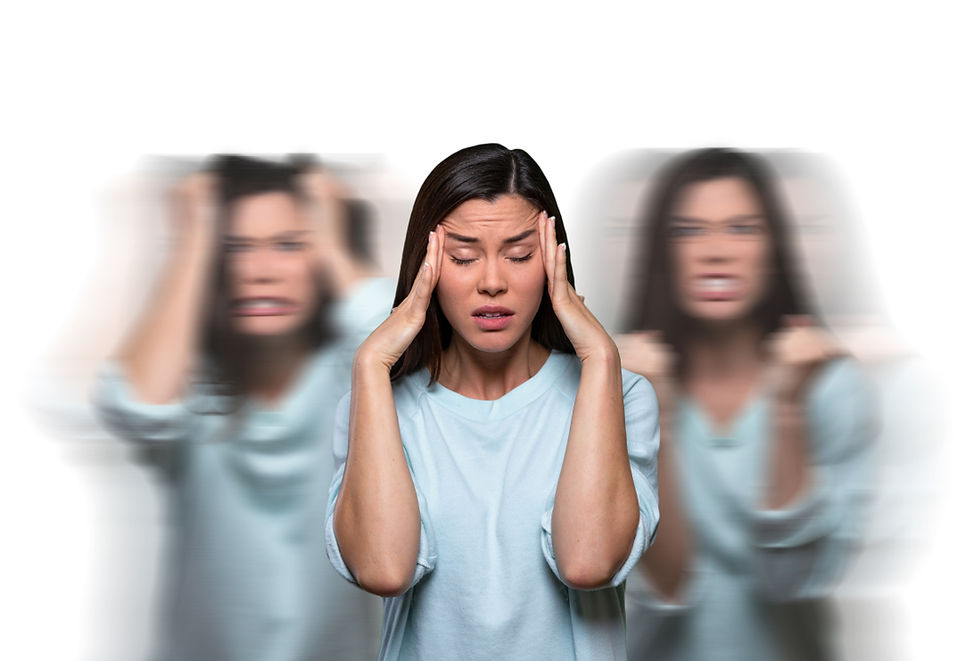
A Reward Deficiency Hypothesis
Before abusing drugs, addicts may require more powerful stimuli (e.g., drugs) to achieve normal enjoyment. In contrast, non-addicts may find drugs too intense to be enjoyable.
After chronic drug use, addicts experience a further weakening of their frontal lobe response to primary reinforcers (e.g., food) and drugs, which become less pleasurable (Advokat et al., 2019).
Chronic drug abuse downregulates dopamine receptors and dopamine production: The 'high' is blunted (Volkow et al., 2010).
Diminished positive reinforcement motivates addicts to engage in more frequent, intense, novel, and risky behaviors.
How Abused Drugs Affect Reward Circuitry
Abused drugs directly or indirectly increase dopamine release at the midbrain ventral tegmental area (VTA), which projects upwards to the forebrain nucleus accumbens, amygdala, hippocampus, and prefrontal cortex. This circuit, called the mesolimbocortical pathway, is also activated by natural rewards (e.g., food and intimacy) and participates in non-drug addictions like pathological gambling (Nestler, 2005; Zhang et al., 2018). Graphic © Fernando Da Cunha/Science Photo Library.
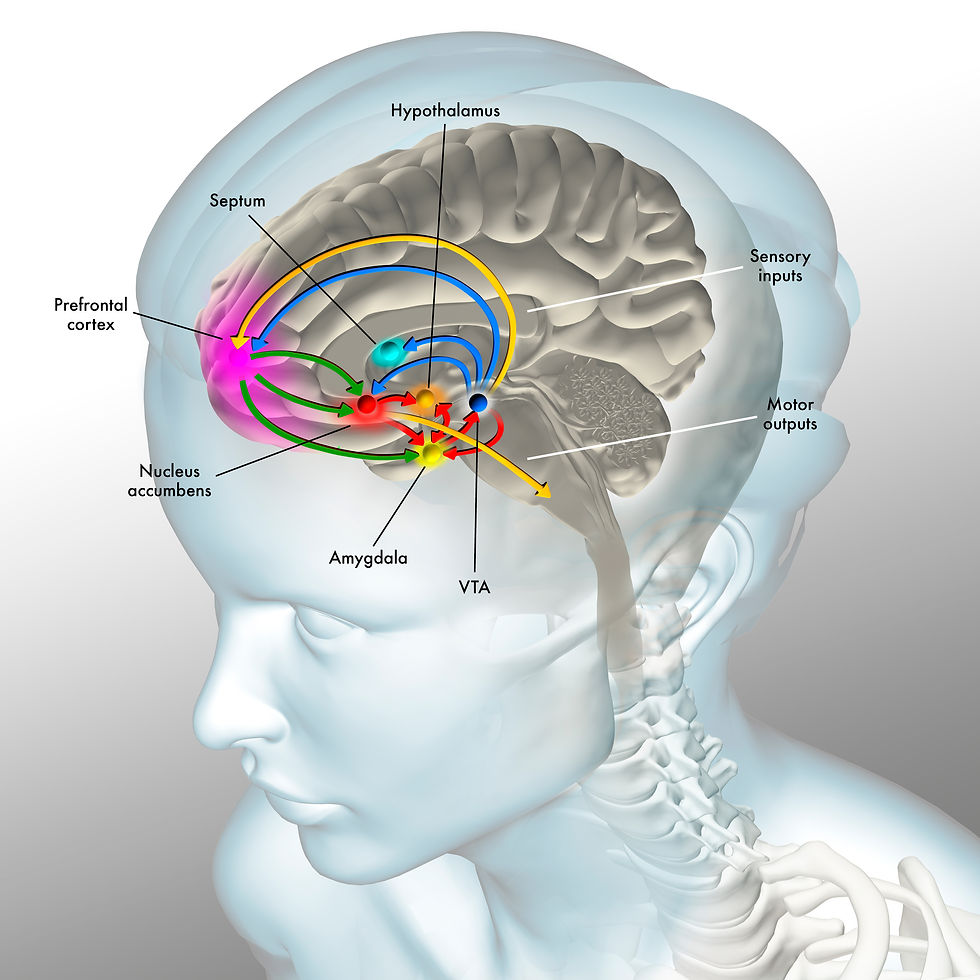
Modified artist's caption. Illustration of the reward pathway in the human brain. This pathway is initiated when a pleasurable stimulus, such as food, sex, or drugs, is experienced. The stimulus (upper orange arrow) triggers the release of dopamine (blue arrows) by the ventral tegmental area (VTA, dark blue sphere). Dopamine travels to several different areas of the brain that, in turn, release dopamine (red arrows). These areas include the nucleus accumbens (red sphere), which initiates motor outputs (lower orange arrow) to sustain the pleasurable stimulus, the prefrontal cortex (pink), which shifts focus and planning toward the pleasurable stimulus, and the amygdala, which is involved in conditioned learning and emotions. Descending prefrontal cortex glutamate projections (green arrows) to the nucleus accumbens and VTA produce craving.
Addicts experience pleasure when VTA axons release large dopamine pulses to bind to the nucleus accumbens, increasing its firing rate. The amygdala classifies drug-related stimuli as salient and creates emotional memories of previous drug use. The hippocampus helps create declarative memories of their use. Finally, the PFC integrates information regarding drug availability, stimulus value, and subjective value (salience). The PFC plans how to obtain and ingest drugs, associates drug cues with craving, reinforces drug use, and inhibits action and regulates emotion (Advokat et al., 2019; George & Koob, 2013; Volkow et al., 2019).
Craving
Craving emerges from a complex network of subcortical and cortical projections. These include the medial hypothalamus (MH) to the medial prefrontal cortex (mPFC) and the DLPFC, the insula to the ACC, OFC, and habenula, and the descending glutamate pathway from the PFC to the VTA and NAC.
The Medial Hypothalamus
The MH may contribute to craving (Gray & Critchley, 2007) through its connections with the NAC and vmPFC.
Cocaine Use Disorder (CUD) is associated with decreased MH-NAC connectivity (Zhang et al., 2018). Self-rated cocaine craving is inversely correlated with communication between these structures. Further research is needed to explain how communication disruption translates into motivated behavior.
In addition, descending vmPFC-MH connectivity is disrupted in CUD, possibly interfering with vmPFC inhibition of craving.
Heightened frontal lobe sensitivity to stressors, drug cues, and the original drug can trigger craving. Any of these sources can contribute to relapse, overwhelming the vmPFC's ability to inhibit drug seeking and ingesting.
The Insula
The insula, particularly the right anterior insula, communicates with the ACC and OFC.
The insula plays a role in nicotine craving and relapse. Animal nicotine research has implicated the insula in relapse following abstinence due to environmental cues (Ghareh et al., 2022). A prospective study of 156 smokers with insula damage due to an ischemic stroke found increased rates of 3-month abstinence (Abdolahi et al., 2015).
During acute nicotine withdrawal, the insula arbitrates bottom-up versus top-down saliency mechanisms to guide behavior – either to pursue smoking or to avoid relapse – and this arbitration is associated with craving and the nicotine withdrawal syndrome (Regner et al., 2019).
The Lateral Habenula
The insula communicates with the habenula and may influence the probability of responding in a given context, such as environmental cues (Khalighinejad et al., 2021).
Moreover, animal and human research supports the role of the lateral habenula in the negative affective changes accompanying withdrawal from addicting drugs (Clerke et al., 2021). Aversive emotional states increase drug cravings and motivate drug relapse.
A Final Common Path for Craving
A descending glutamate pathway from the PFC to the VTA and nucleus accumbens plays a crucial role in drug craving (Wanchoo et al., 2009). Chronic exposure to abused drugs (alcohol, amphetamines, benzodiazepines, cocaine, morphine, and nicotine) increases glutamate release at the VTA and nucleus accumbens (Madsen et al., 2012).
Liking and Wanting
In healthy individuals, there is a balance between liking and wanting. However, in substance use disorder, liking decreases due to D2 receptor downregulation, and wanting (i.e., craving) increases. This hedonic shift promotes compulsive drug use and relapse. Imagine a chain smoker who compulsively lights cigarette after cigarette without experiencing enjoyment.
Mediating Neurotransmitters
Although dopamine begins the neurotransmitter cascade, mediating neurotransmitters include acetylcholine, endocannabinoids, endogenous opioids, GABA, glutamate, glycine, and serotonin.
Processes That Promote Cell Death in the Cortex and Limbic System
The loss of cortical and limbic neurons contributes to hypofrontality, which involves reduced PFC perfusion, metabolic activity, and performance of executive functions. This compromises the vmPFC's ability to resist craving and promotes relapse. An addict's brain may not recover after 12 months of sobriety.
Chronic drug abuse can contribute to apoptosis or programmed cell death. Drugs like methamphetamine are neurotoxins that target neurons that release dopamine and serotonin.
Moreover, the stress produced by chronic substance use disorder can cause chronic elevations of the stress hormone cortisol. High sustained cortisol levels are also neurotoxic, especially to hippocampal neurons concerned with declarative memory, regulating endocrine stress responses, and processing sensory information.
Cortisol interferes with repairing damaged neurons via proteins like BDNF and neurogenesis, creating new neurons. Neurogenesis occurs in the hippocampus and possibly the cortex in adult humans.
Methamphetamine devastates networks involved in emotion and reward (limbic system), executive functions (PFC), and memory (hippocampus).
A compromised prefrontal cortex suffers from impaired long-term planning, decision-making, and moral judgment. Graphic © SciePro/Shutterstock.com.
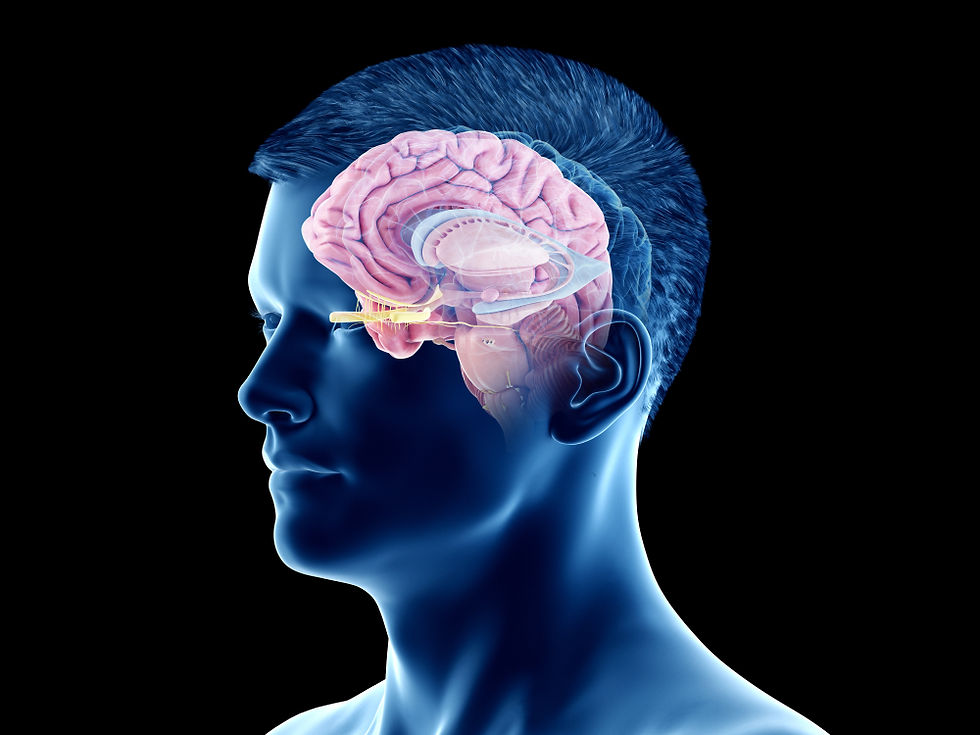
Brain recovery depends on the individual and their drug use history. For cocaine use disorder, full recovery of these functions may not occur after 4 months of drug abstinence (Advokat et al., 2021).
Magnetic resonance imaging (MRI) revealed that adult addicts had less cortical volume than controls one week and 12 months after abstinence. Addicts with the lowest cortical volumes and surface area were at greater risk for relapsing after one year (Durazzo et al., 2011).
Hypofrontality Promotes Relapse
The loss of prefrontal brain volume can produce a phenomenon called hypofrontality, in which PFC metabolic activity and the performance of executive functions are compromised. Hypofrontality reduces the vmPFC's ability to resist craving, anticipate consequences, and inhibit risky behavior. Hypofrontality underlies loss of control (ventromedial PFC) and relapse.
Executive functions include the cognitive processes that control attention, behavior inhibition, working memory, cognitive flexibility, reasoning, problem-solving, and planning (Breedlove & Watson, 2020). The inattentive subtype of ADHD involves impaired continuous attention. Graphic © Oksana Mizina/Shutterstock.com.
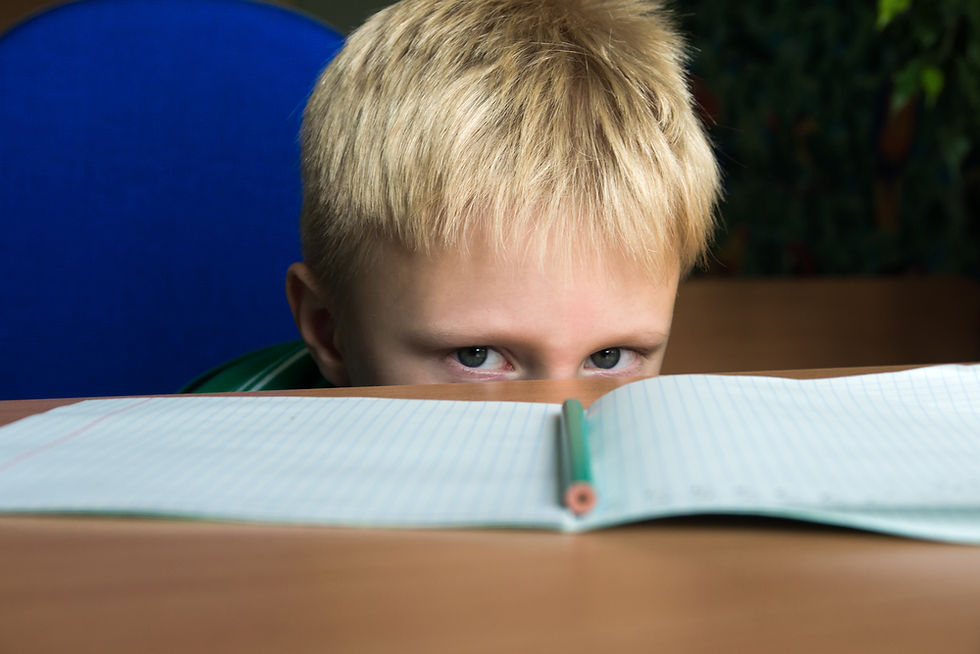
Neurofeedback Efficacy in Substance Use Disorder
Neurofeedback is an evidence-based treatment for substance use disorder. Sokhadze and Trudeau (in press) assigned a level-3 rating of probably efficacious to the Scott-Kaiser modification of the Peniston Protocol plus rehabilitation for substance use disorder (SUD). The authors based their findings on six randomized controlled trials, one quasi-experimental study, and four case studies of alcohol, opiate, stimulant, and mixed SUDs.
The Scott-Kaiser (1998) approach combines training to increase attention (e.g., reinforcing beta and/or SMR while suppressing theta) with Peniston's (Peniston & Kulkosky, 1989, 1990, 1991) alpha-theta training.

Summary
A comprehensive model of addiction combines negative and positive reinforcement. Substance use disorder encompasses craving and loss of reinforcement. The orbitofrontal cortex, anterior cingulate cortex, and nucleus accumbens process craving-related information in response to drug-related cues. The ventromedial prefrontal cortex plays a key role in emotional control and inhibiting actions associated with deleterious consequences. Although many factors contribute to developing substance use disorders, stress and deficient dopamine receptors may be crucial. Addiction involves diverse neurotransmitters, including dopamine, endocannabinoids, endogenous opioids, GABA, glycine, and glutamate. Drug damage to neurons and glial cells, interference with repair, and the suppression of neurogenesis cause hypofrontality and impaired behavioral and emotional control. Neurofeedback is a promising substance use disorder intervention. The Scott-Kaiser modification of the Peniston Protocol plus rehabilitation for substance use disorder has achieved level-3 efficacy based on six randomized controlled trials, one quasi-experimental study, and four case studies.
Glossary
alpha-theta training: a protocol to slow the EEG to the 6-9 Hz crossover region while maintaining alertness.
anterior cingulate cortex (ACC): a part of the prefrontal cortex that plays an important role in drug craving and the Go system that predominates during the preoccupation/anticipation stage of addiction. The ACC plays an important role in attention and working memory and mediates emotional and physical pain.
apoptosis: programmed cell death.
bed nucleus of the stria terminalis (BNST): a complex hub connecting the amygdala, hippocampus, and prefrontal cortex to orchestrate emotional and behavioral stress responses and modulate craving.
central nucleus of the amygdala (CeA): a nucleus that orchestrates the nervous system's response to important stimuli by activating circuits in the brainstem (autonomic arousal) and the basal ganglia and periaqueductal gray (defensive behavior).
cortisol: a glucocorticoid produced by the adrenal cortex that helps convert fat and protein to glucose, reduces inflammation, and participates in apoptosis.
craving: intense motivation to acquire and ingest a drug, often triggered by cues.
D1 and D2 dopamine receptors: two types of receptors in the nucleus accumbens involved in dopamine signaling; D1 receptors are typically associated with reward, while D2 receptors are often linked to aversive responses.
dopamine: monoamine neurotransmitter released by the ventral tegmental area in response to natural reinforcers (e.g., food or sex) or self-administering a drug.
dorsolateral prefrontal cortex (DLPFC): the DLPFC is concerned with executive functioning and regulating cognitive processes. DLPFC activation promotes craving by intensifying the response to drug-related cues.
executive functions: the cognitive processes that control attention, inhibition of behavior, working memory, cognitive flexibility, reasoning, problem-solving, and planning
globus pallidus (GP): a basal ganglia nucleus that smooths voluntary movements through subconscious inhibition, countering the cerebellum's excitatory influence. The GP enables the NAC to act in response to craving.
glutamate: an amino acid neurotransmitter that promotes craving when released by descending prefrontal cortex pathways targeting the nucleus accumbens and ventral tegmental area.
habenula: dorsal diencephalic nucleus involved in reward and aversive processing.
hippocampus (HPC): a limbic structure in the medial temporal lobe involved in 4-7 Hz theta activity, control of the endocrine system’s response to stressors, formation of explicit memories, and navigation. The HPC influences craving by providing affective state, context, and stress information.
hypofrontality: reduced PFC perfusion, metabolic activity, and performance of executive functions. Hypofrontality reduces the frontal lobe's ability to resist craving, anticipate consequences, and inhibit risky behavior.
insula: pyramid-shaped cortex deep within the lateral sulcus, called the island of Reil.
limbic system: a poorly-defined widespread network of nuclei involved in emotion, motivation, learning, memory, and navigation. Three important limbic structures are the hippocampus, amygdala, and septal nuclei. The limbic system influences craving by providing affective state information.
medial orbitofrontal cortex (mOFC): a prefrontal cortex subdivision that monitors the reward value of reinforcers and mediates learning and memory about our reinforcement experiences.
mTOR pathway: a key signaling pathway involved in cell growth, protein synthesis, and neural plasticity, which drugs of abuse exploit to alter brain circuits.
negative reinforcement: strengthening operant behaviors that enable avoidance or escaping aversive stimuli (e.g., a dysphoric state due to drug withdrawal).
neural stem cells: multipotent cells that can differentiate into neurons, astrocytes, and oligodendrocytes.
neurogenesis: creation of new neurons from stem cells in the cortex and hippocampus.
nucleus accumbens (NAC): a ventral striatal structure targeted by dopamine released by the mesolimbic pathway. The NAC is involved in acute pleasure, reward, and the Go system.
orbitofrontal cortex (OFC): a frontal lobe subdivision that integrates sensory information, modulates visceral reactions, and participates in learning, predicting outcomes, and making choices regarding emotional and reward-related behaviors. the frontal lobe subdivision involved in the Stop system and craving. The OFC decodes stimuli's punishment and reward value and helps inhibit inappropriate behavior.
Peniston Protocol: a multimodal approach that incorporates visualization training, temperature biofeedback, rhythmic breathing techniques, autogenic training exercises, construction of personalized imagery, guided imagery, and 30 alpha-theta sessions.
punishment: the weakening of operant behaviors by aversive consequences.
rheb: a protein involved in the mTOR signaling pathway; crucial for drugs of abuse to hijack natural reward pathways in the NAc and suppress responses to physiological needs.
salient stimuli: events that we prioritize.
Scott-Kaiser modification of the Peniston Protocol: training to increase attention (e.g., reinforcing beta and/or SMR while suppressing theta) with Peniston's alpha-theta training.
substance use disorders (SUDs): treatable, chronic diseases characterized by substance use that impairs health, social function, and behavioral control. Individuals continue substance use despite harmful consequences to themselves and others (CDC).
ventral tegmental area (VTA): the midbrain hub of the mesolimbocortical pathway that plays a significant role in aversion, cognition, motivation, and reward. Ascending VTA neurons release dopamine and GABA.
ventromedial prefrontal cortex (vmPFC): a frontal lobe subdivision involved in emotional control, inhibition of action, empathy, episodic and semantic memory, and economic decision-making. Hypofrontality of the vmPFC renders the brain more vulnerable to cue-induced craving and drug use.
References
Abdolahi, A., Williams, G. C., Benesch, C. G., Wang, H. Z., Spitzer, E. M., Scott, B. E., Block, R. C., & van Wijngaarden, E. (2015). Smoking cessation behaviors three months following acute insular damage from stroke. Addictive behaviors, 51, 24–30. https://doi.org/10.1016/j.addbeh.2015.07.001 Advokat, C. D., Comaty, J. E., & Julien, R. M. (2019). Julien's primer of drug action: A comprehensive guide to the actions, uses, and side effects of psychoactive drugs (14th ed.). Worth Publishers. Bouarab, C., Thompson, B., & Polter, A. M. (2019). VTA GABA neurons at the interface of stress and reward. Frontiers in neural circuits, 13, 78. https://doi.org/10.3389/fncir.2019.00078
Clerke, J. A., Congiu, M., & Mameli, M. (2021). Neuronal adaptations in the lateral habenula during drug withdrawal: Preclinical evidence for addiction therapy. Neuropharmacology, 192, 108617. https://doi.org/10.1016/j.neuropharm.2021.108617
Durazzo, T. C., Tosun, D., Buckley, S., Gazdzinski, S., Mon, A., Fryer, S. L., & Meyerhoff, D. J. (2011). Cortical thickness, surface area, and volume of the brain reward system in alcohol dependence: Relationships to relapse and extended abstinence. Alcoholism, Clinical and Experimental Research, 35(6), 1187–1200. https://doi.org/10.1111/j.1530-0277.2011.01452.x
Ghareh, H., Alonso-Lozares, I., Schetters, D., Herman, R. J., Heistek, T. S., Van Mourik, Y., Jean-Richard-Dit-Bressel, P., Zernig, G., Mansvelder, H. D., De Vries, T. J., & Marchant, N. J. (2022). Role of anterior insula cortex in context-induced relapse of nicotine-seeking. eLife, 11, e75609. https://doi.org/10.7554/eLife.75609
Gray, M. A., & Critchley, H. D. (2007). Interoceptive basis to craving. Neuron, 54(2), 183–186. https://doi.org/10.1016/j.neuron.2007.03.024
Haass-Koffler, C. L., & Bartlett, S. E. (2012). Stress and addiction: Contribution of the corticotropin releasing factor (CRF) system in neuroplasticity. Frontiers in Molecular Neuroscience, 5, 91. https://doi.org/10.3389/fnmol.2012.00091 Hayashi, T., Ko, J. H., Strafella, A. P., & Dagher, A. (2013). Dorsolateral prefrontal and orbitofrontal cortex interactions during self-control of cigarette craving. Proceedings of the National Academy of Sciences of the United States of America, 110(11), 4422–4427. https://doi.org/10.1073/pnas.1212185110
Khalighinejad, N., Garrett, N., Priestley, L., Lockwood, P., & Rushworth, M. F. S. (2021). A habenula-insular circuit encodes the willingness to act. Nature Communications, 12(1), 6329. https://doi.org/10.1038/s41467-021-26569-1
Koob, G. F. (2013). Addiction is a reward deficit and stress surfeit disorder. Frontiers in Psychiatry, 4, 72. https://doi.org/10.3389/fpsyt.2013.00072
Madsen, H. B., Brown, R. M., & Lawrence, A. J. (2012). Neuroplasticity in addiction: Cellular and transcriptional perspectives. Frontiers in Molecular Neuroscience, 5, 99. https://doi.org/10.3389/fnmol.2012.00099
Nestler E. J. (2005). Is there a common molecular pathway for addiction?. Nature Neuroscience, 8(11), 1445–1449. https://doi.org/10.1038/nn1578
Nguyen, D., Naffziger, E. E., & Berridge, K. C. (2021). Positive Affect: Nature and brain bases of liking and wanting. Current Opinion in Behavioral Sciences, 39, 72–78. https://doi.org/10.1016/j.cobeha.2021.02.013
Peniston, E. G., & Kulkosky, P. J. (1989). Alpha-theta brainwave training and beta endorphin levels in alcoholics. Alcoholism Clinical and Experimental Research, 13, 271–279.
Peniston, E. G., & Kulkosky, P. J. (1990). Alcoholic personality and alpha-theta brainwave training. Medical Psychotherapy, 2, 37–55.
Peniston, E. G., & Kulkosky, P. G. (1991). Alpha-theta brain wave neurofeedback for Vietnam veterans with combat related post traumatic stress disorder. Medical Psychotherapy, 4, 1–14.
Regner, M. F., Tregellas, J., Kluger, B., Wylie, K., Gowin, J. L., & Tanabe, J. (2019). The insula in nicotine use disorder: Functional neuroimaging and implications for neuromodulation. Neuroscience and Biobehavioral Reviews, 103, 414–424. https://doi.org/10.1016/j.neubiorev.2019.06.002
Scott, W., & Kaiser, D. (1998). Augmenting chemical dependency treatment with neurofeedback training. Journal of Neurotherapy, 3(1), 66.
Scott, W. C., Kaiser, D., Othmer, S., & Sideroff, S. I. (2005). Effects of an EEG biofeedback protocol on a mixed substance abusing population. American Journal of Drug Alcohol Abuse, 31(3), 455–469.
Sokhadze, E. M., & Trudeau, D. L. (in press). Alcohol and drug dependence. In I. Z. Khazan, F. Shaffer, D. Moss, R. Lyle, & S. Rosenthal (Eds.). Evidence-based practice in biofeedback and neurofeedback (4th ed.). Association for Applied Psychophysiology and Biofeedback.
Tan, B., Browne, C. J., Nöbauer, T., Vaziri, A., Friedman, J. M., & Nestler, E. J. (2023). Drugs of abuse hijack a mesolimbic pathway that processes homeostatic need. bioRxiv: the preprint server for biology, 2023.09.03.556059. https://doi.org/10.1101/2023.09.03.556059
Volkow, N., & Boyle, M. (2018). Neuroscience of addiction: Relevance to prevention and treatment. The American Journal of Psychiatry, 175(8), 729-740. https://doi.org/10.1176/appi.ajp.2018.17101174
Volkow, N. D., Wang, G. J., Fowler, J. S., Tomasi, D., Telang, F., & Baler, R. (2010). Addiction: Decreased reward sensitivity and increased expectation sensitivity conspire to overwhelm the brain's control circuit. BioEssays: News and Reviews in Molecular, Cellular and Developmental Biology, 32(9), 748–755. https://doi.org/10.1002/bies.201000042
Wanchoo, S. J., Swann, A. C., & Dafny, N. (2009). Descending glutamatergic pathways of PFC are involved in acute and chronic action of methylphenidate. Brain Research, 1301, 68–79. https://doi.org/10.1016/j.brainres.2009.08.095
Zhang, S., Wang, W., Zhornitsky, S., & Li, C. R. (2018). Resting state functional connectivity of the lateral and medial hypothalamus in cocaine dependence: An exploratory study. Frontiers in Psychiatry, 9, 344. https://doi.org/10.3389/fpsyt.2018.00344
Support Our Friends
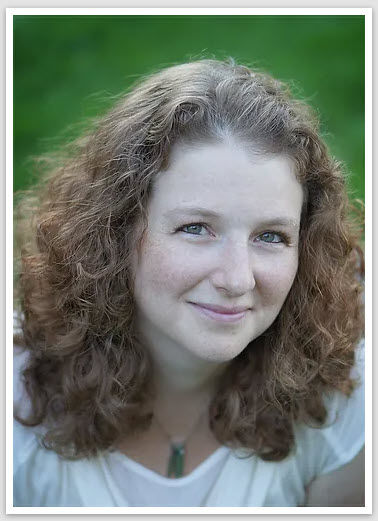
Dr. Inna Khazan's BCIA Introduction to biofeedback workshop will be offered in two parts this year.
Part 1 is entirely virtual, consisting of 20 hours (over 5 days) of live online instruction, home-study materials distributed prior to the live workshop, and written instructions for practical lab work to be completed during the week of the workshop or after its completion. Part 1 fulfills BCIA requirements for introduction to biofeedback didactic. Part 1 will take place on Zoom, November 4 - 8, 2024, 12 - 4pm EDT. Tuition is $1395.
Part 2 is optional, and consists of 14 hours (over 2 days) of in-person hands-on practical training using state-of-the-art equipment, designed to help participants be better prepared to start working with clients. Part 2 will take place in Boston on November 11 & 12, 2024, 9am-5pm EDT. Tuition is $395. (Please note that an Introduction to Biofeedback didactic (taken at any previous time, anywhere) is a pre-requisite to the hands-on training).
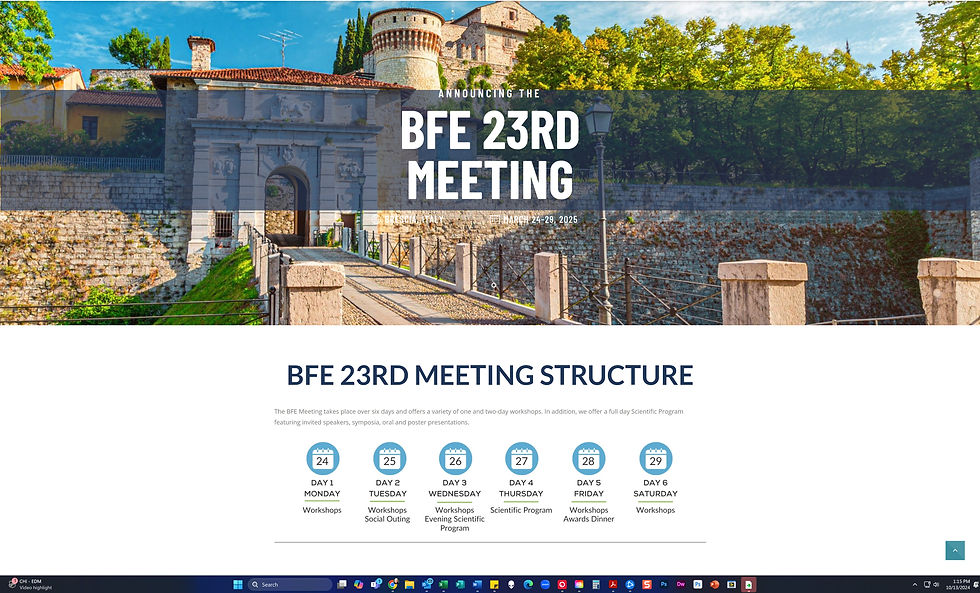

2. loss of time anchors (e.g., commuting to work)
Comments