5-Min Science: EEG Traveling Waves
- BioSource Faculty
- 2 days ago
- 8 min read
Updated: 23 hours ago

Traveling waves in electroencephalography (EEG) represent coordinated patterns of electrical activity that move systematically across the cortex.
Instead of being static or localized, these waves propagate spatially, carrying neural signals from one cortical region to another. Historically, EEG analysis focused heavily on localized brain oscillations and synchronous rhythms within confined cortical areas. However, recent advancements in signal processing and neuroimaging techniques have highlighted traveling waves as crucial for understanding dynamic cortical connectivity (Muller et al., 2018). Traveling waves challenge traditional assumptions of stationary EEG activity, thereby reshaping how neuroscientists interpret brain wave patterns in both health and disease.
Mechanisms and Origins of Traveling Waves
Traveling waves originate from oscillatory activity generated by synchronized neuronal populations, typically driven by excitatory-inhibitory neuronal interactions. Cortical and subcortical structures, including the thalamus, are integral in initiating and propagating these waves. The spatiotemporal dynamics of traveling waves depend upon precise synaptic connections, cortical layers, and neurotransmitter balances, especially glutamate and gamma-aminobutyric acid (GABA).
For instance, alpha-frequency traveling waves (~8–12 Hz) arise prominently in posterior regions and propagate anteriorly, reflecting functional connectivity between visual and frontal executive networks (Halgren et al., 2019). Understanding these mechanisms enhances the clinician’s and researcher’s ability to interpret EEG signals more meaningfully.
Traveling Waves in Perception and Attention
Alpha-band (8–12 Hz) traveling waves play a key role in visual attention and sensory processing. Visual stimuli can evoke traveling alpha waves that propagate from posterior to anterior regions, modulating sensory gain and attention allocation (Lozano-Soldevilla & VanRullen, 2019). These waves dynamically gate sensory inputs by rhythmically facilitating or suppressing cortical excitability. Aberrant alpha traveling-wave dynamics have been associated with impaired attentional control and distractibility, frequently observed in ADHD and mild cognitive impairment (Muller et al., 2018).
Traveling Waves in Cognition
Traveling waves facilitate cognitive processes by supporting communication between disparate cortical areas. For example, theta-frequency (~4–7 Hz) traveling waves are essential for memory encoding and retrieval, helping coordinate activity between the hippocampus and cortical regions involved in memory formation (Zhang et al., 2018). Similarly, alpha-band traveling waves modulate attention by selectively enhancing or suppressing sensory input depending on task demands. By guiding synchronized activity across networks, traveling waves thus act as dynamic conductors, influencing which neural populations communicate at a given moment (Massimini et al., 2004).
Traveling Waves in Neural Binding
Traveling waves significantly influence neural binding, the process by which the brain integrates distributed neural activities into coherent perceptions. Gamma-band traveling waves (approximately 30–80 Hz) exemplify this principle. These high-frequency waves propagate across cortical areas, coordinating synchronous neural firing to bind separate perceptual features—such as color, shape, and motion—into a unified perceptual experience (Alexander et al., 2013). Studies demonstrate that disruptions or abnormalities in gamma-wave propagation patterns may underlie perceptual deficits and cognitive dysfunction in schizophrenia, autism spectrum disorder, and other disorders characterized by impaired sensory integration (Lozano-Soldevilla & VanRullen, 2019).
Traveling Waves in Sleep
Traveling waves are fundamental to the organization and restorative functions of sleep. A classic example is the slow oscillation (<1 Hz) prominent during non-rapid eye movement (NREM) sleep, characterized by rhythmic depolarization (up-states) and hyperpolarization (down-states) of cortical neurons propagating systematically across the cortex (Massimini et al., 2004). These waves typically originate in frontal regions and propagate posteriorly, coordinating neuronal activity crucial for memory consolidation and synaptic plasticity (Muller et al., 2018). Disruption of slow-wave propagation patterns during sleep has been associated with impaired memory consolidation, cognitive decline, and psychiatric conditions like depression (Halgren et al., 2019).
Another sleep-related example is the sleep spindle (12–16 Hz), which also demonstrates traveling-wave behavior. Spindles often propagate from central to frontal cortical areas, synchronizing neuronal firing across the cortex and thalamus, and facilitating memory processing and integration. Changes in spindle propagation patterns have been linked to cognitive impairment and aging, underscoring the clinical relevance of traveling-wave dynamics during sleep (Muller et al., 2018).
Detection and Analysis Techniques in EEG
Detecting traveling waves involves advanced analytical techniques beyond conventional spectral analysis. Traditional EEG approaches often overlook propagation because they focus exclusively on local power changes. Modern approaches, such as phase-gradient analysis, enable researchers to quantify the speed, directionality, and spatial coherence of EEG traveling waves. These methods utilize phase differences across electrode arrays to map cortical wave trajectories. High-density EEG arrays provide the spatial resolution necessary to accurately track wave propagation across cortical regions, overcoming limitations of standard low-density recordings (Alexander et al., 2013).
Importance for Quantitative EEG (qEEG)
Quantitative EEG (qEEG) traditionally employs power spectral analysis to compare brainwave activity against normative databases. The incorporation of traveling-wave analysis significantly enriches the qEEG’s diagnostic and interpretive power. Recognizing traveling wave abnormalities can help identify disrupted cortical connectivity patterns associated with psychiatric and neurological disorders. For example, altered alpha traveling-wave propagation patterns have been associated with attention deficit hyperactivity disorder (ADHD), schizophrenia, and Alzheimer's disease, adding a new dimension to biomarker identification and clinical assessment (Lozano-Soldevilla & VanRullen, 2019).
Traveling Waves in Clinical Neurofeedback
In neurofeedback, patients train to modulate brain activity by receiving real-time feedback from their EEG. Historically, neurofeedback protocols have targeted localized frequency power changes (e.g., alpha amplitude enhancement). Integrating traveling wave dynamics into neurofeedback represents a novel therapeutic avenue. By training the directional propagation or coherence of traveling waves, neurofeedback practitioners could potentially enhance cortical integration or normalize aberrant connectivity. Preliminary findings suggest this method could improve outcomes in disorders involving widespread network disruptions, such as epilepsy or traumatic brain injury (Breakspear, 2017).
Relevance to Neurodevelopmental Disorders
Traveling waves are particularly informative in neurodevelopmental disorders, where large-scale cortical connectivity disruptions frequently occur. For example, research indicates that individuals with autism spectrum disorder (ASD) exhibit atypical traveling wave propagation patterns in the alpha and theta bands, reflecting underlying cortical dysconnectivity (Roberts et al., 2019). Likewise, dysregulated propagation speed or abnormal spatial trajectories of traveling waves have been observed in developmental disorders like ADHD, potentially explaining symptoms related to executive dysfunction and attention deficits. Detecting and characterizing these traveling wave disruptions may thus enhance diagnostic precision and intervention targeting.
Applications in Neurological and Psychiatric Disorders
Beyond neurodevelopmental disorders, altered traveling waves are evident in neurological and psychiatric conditions such as epilepsy, major depressive disorder (MDD), and schizophrenia. In epilepsy, traveling wave analysis can identify epileptogenic zones, improve surgical outcomes, and guide personalized neurostimulation therapies (Muller et al., 2018). Similarly, disrupted alpha and delta traveling waves in MDD correlate with symptom severity and response to antidepressants, offering potential predictive biomarkers. In schizophrenia, aberrant gamma traveling waves reflect disturbances in perceptual integration and cognitive processing, informing targeted neurofeedback interventions (Davis et al., 2020).
Challenges and Future Directions
Despite their promise, traveling waves in EEG face several methodological and interpretive challenges. Accurate detection requires dense EEG arrays and computationally intensive analyses, making routine clinical use complex. Furthermore, translating basic science findings into practical neurofeedback protocols remains an ongoing research frontier. Future directions involve developing standardized metrics for clinical traveling wave analysis, validating normative databases, and integrating wave dynamics with established qEEG parameters. Advances in machine learning and artificial intelligence will likely simplify detection methods and support real-time neurofeedback training based on traveling wave modulation.
Conclusion: The Promise of Traveling Waves in EEG
Traveling waves represent a dynamic and essential mechanism by which the brain coordinates neuronal activity across extensive cortical regions. Far from being static or localized phenomena, these waves actively facilitate key functions, including memory consolidation during sleep, integration of perceptual features through neural binding, and modulation of attention and sensory processing. Their relevance spans cognitive, psychiatric, and neurological domains, providing novel insights and clinical biomarkers for conditions such as epilepsy, autism, ADHD, depression, and schizophrenia. Incorporating traveling wave analyses into quantitative EEG (qEEG) significantly enhances diagnostic capabilities, enabling clinicians to detect subtle disruptions in brain connectivity patterns not captured by traditional EEG methods alone.
Moreover, traveling waves open innovative avenues for neurofeedback interventions, moving beyond conventional approaches focused solely on amplitude modulation. Training patients to normalize aberrant traveling wave dynamics could yield substantial therapeutic benefits, particularly for disorders characterized by disrupted cortical integration or altered connectivity. Although methodological challenges remain, ongoing advancements in computational techniques, high-density EEG technology, and machine learning promise to simplify detection and facilitate clinical translation. As research progresses, traveling waves are likely to become central to personalized approaches in EEG diagnostics and neurotherapy, fundamentally reshaping our understanding and treatment of brain dysfunction.
Key Takeaways
Traveling waves are dynamic EEG patterns that propagate systematically across the cortex, supporting large-scale neural communication essential for cognitive processes, including memory, attention, perception, and sensory integration.
Sleep-related traveling waves, such as slow oscillations and sleep spindles, play critical roles in memory consolidation and cortical restoration; disruptions in their propagation patterns correlate with cognitive deficits and neuropsychiatric disorders.
Gamma-band traveling waves facilitate neural binding, integrating distributed cortical signals into coherent perceptions; abnormalities in gamma-wave propagation are linked to perceptual and cognitive dysfunctions in schizophrenia and autism spectrum disorder.
Advanced analytical methods like phase-gradient analysis and high-density EEG arrays are necessary for accurate detection and characterization of traveling waves, enhancing the diagnostic power of quantitative EEG (qEEG).
Incorporating traveling wave dynamics into neurofeedback protocols offers promising therapeutic potential by targeting network-wide cortical dysfunctions, opening novel treatment possibilities for psychiatric and neurological disorders involving impaired connectivity.
Glossary
alpha rhythm: oscillations in the EEG around 8–12 Hz, typically dominant over the occipital cortex during relaxed wakefulness.
coherence: a measure of synchrony or similarity between electrical signals recorded from different EEG sites, reflecting functional connectivity.
cortical connectivity: the functional relationships and communication pathways between different regions of the cerebral cortex.
delta rhythm: EEG oscillations at frequencies ≤ 4 Hz, commonly observed during deep sleep or pathological brain states.
electroencephalography (EEG): the non-invasive recording of electrical activity from the brain using electrodes placed on the scalp.
gamma-aminobutyric acid (GABA): the principal inhibitory neurotransmitter in the brain, crucial for modulating neuronal excitability and cortical oscillations.
high-density EEG array: EEG recording setup involving numerous closely spaced electrodes, providing detailed spatial resolution to detect traveling waves and subtle neural dynamics.
machine learning: computational methods that allow algorithms to identify complex patterns in EEG data, useful for diagnostic prediction and neurofeedback guidance.
neural binding: the process by which the brain integrates separate features of sensory inputs (such as color, shape, sound, or motion), which are initially processed in different cortical regions, into a single unified perception. Binding occurs via synchronized neuronal activity, often reflected as coordinated high-frequency (gamma-band) oscillations traveling across the cortex.
phase-gradient analysis: an analytical technique that determines the direction and speed of EEG traveling waves by evaluating spatial phase differences across electrodes.
quantitative EEG (qEEG): EEG methodology involving mathematical analysis of brainwave data, comparing individuals’ EEG parameters against normative databases for diagnostic or therapeutic purposes.
theta rhythm: EEG oscillations at frequencies around 4–7 Hz, frequently associated with memory processing, emotional regulation, and cognitive control.
traveling waves: coordinated patterns of brain activity that propagate systematically across the cerebral cortex, reflecting large-scale neuronal communication.
References
Alexander, D. M., Trengove, C., Johnston, P., Cooper, T., August, J. P., & Gordon, E. (2013). High-density EEG mapping of cortical travel wave dynamics. Journal of Neuroscience Methods, 217(1–2), 113–122. https://doi.org/10.1016/j.jneumeth.2013.04.014
Breakspear, M. (2017). Dynamic models of large-scale brain activity. Nature Neuroscience, 20(3), 340–352. https://doi.org/10.1038/nn.4497
Davis, Z. W., Muller, L., & Reynolds, J. H. (2020). Traveling waves in cortex: New insights into mechanisms and functions. Frontiers in Systems Neuroscience, 14, 59. https://doi.org/10.3389/fnsys.2020.00059
Halgren, M., Devinsky, O., Doyle, W. K., Bastuji, H., Rey, M., Mak-McCully, R.,... & Cash, S. S. (2019). The generation and propagation of the human alpha rhythm. Proceedings of the National Academy of Sciences, 116(47), 23772–23782. https://doi.org/10.1073/pnas.1913092116
Lozano-Soldevilla, D., & VanRullen, R. (2019). The hidden spatial dimension of alpha: 10-Hz perceptual echoes propagate as periodic traveling waves in the human brain. Cell Reports, 26(2), 374–380. https://doi.org/10.1016/j.celrep.2018.12.058
Massimini, M., Huber, R., Ferrarelli, F., Hill, S., & Tononi, G. (2004). The sleep slow oscillation as a traveling wave. Journal of Neuroscience, 24(31), 6862–6870. https://doi.org/10.1523/JNEUROSCI.1318-04.2004
Muller, L., Chavane, F., Reynolds, J., & Sejnowski, T. J. (2018). Cortical traveling waves: mechanisms and computational principles. Nature Reviews Neuroscience, 19(5), 255–268. https://doi.org/10.1038/nrn.2018.20
Roberts, J. A., Gollo, L. L., Abeysuriya, R. G., Roberts, G., Mitchell, P. B., Woolrich, M. W., & Breakspear, M. (2019). Metastable brain waves. Nature Communications, 10(1), 1056. https://doi.org/10.1038/s41467-019-08999-0
Uhlhaas, P. J., & Singer, W. (2006). Neural synchrony in brain disorders: Relevance for cognitive dysfunctions and pathophysiology. Neuron, 52(1), 155–168. https://doi.org/10.1016/j.neuron.2006.09.020
Zhang, H., Watrous, A. J., Patel, A., & Jacobs, J. (2018). Theta and alpha oscillations are traveling waves in the human neocortex. Neuron, 98(6), 1269–1281. https://doi.org/10.1016/j.neuron.2018.05.019
Support Our Friends
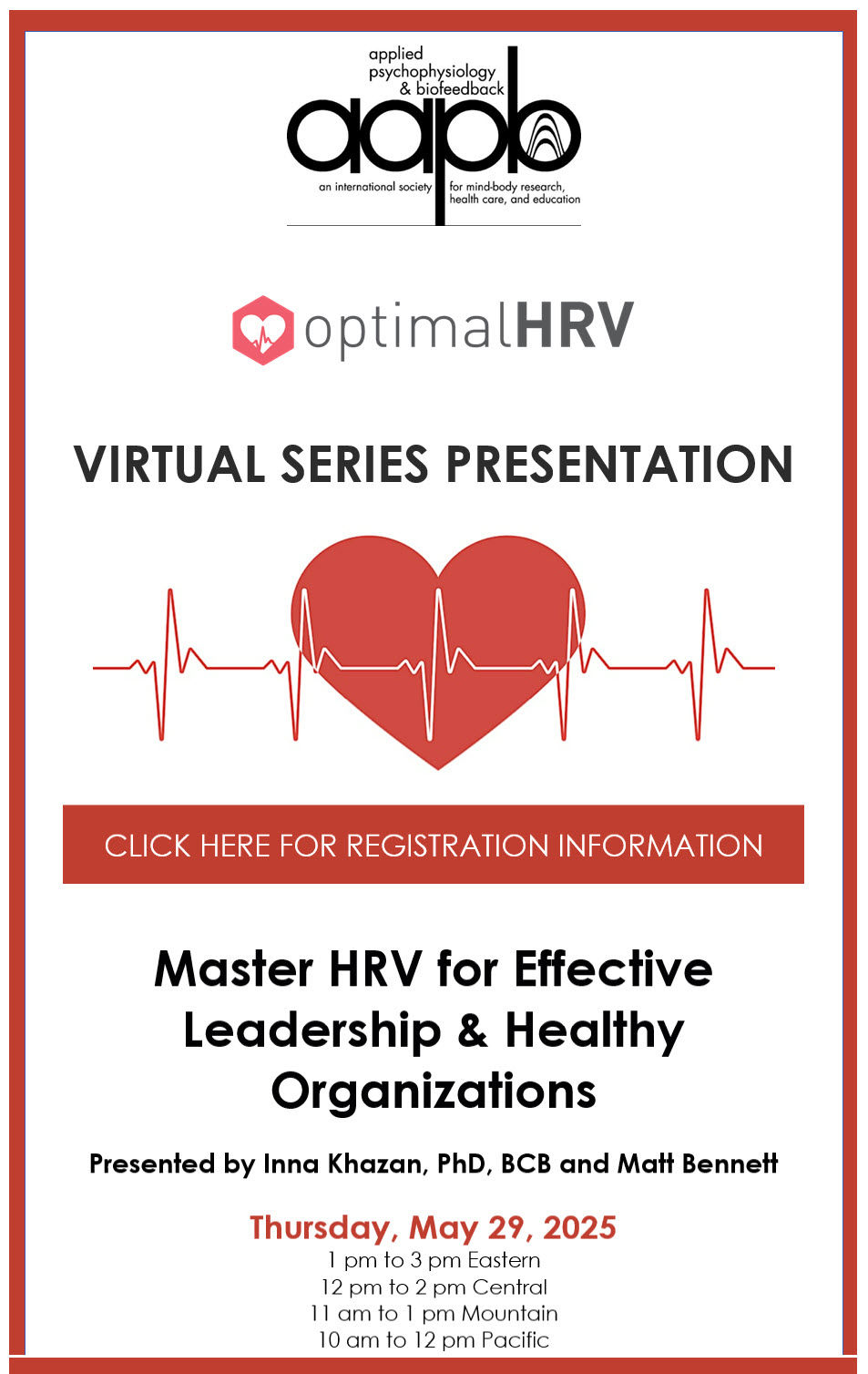

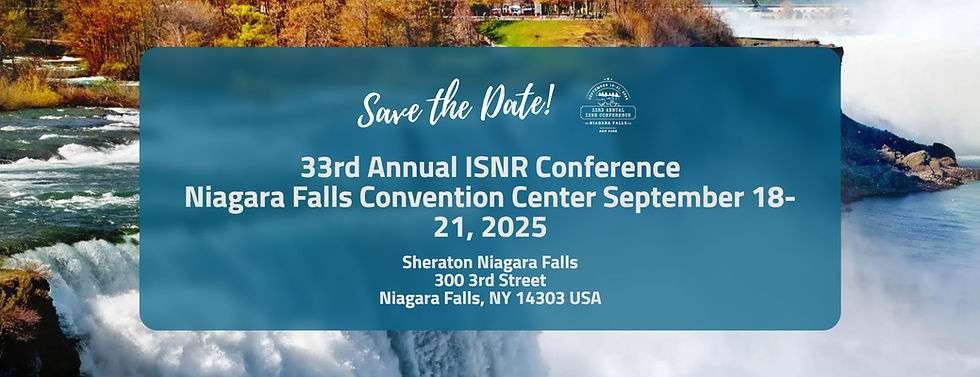
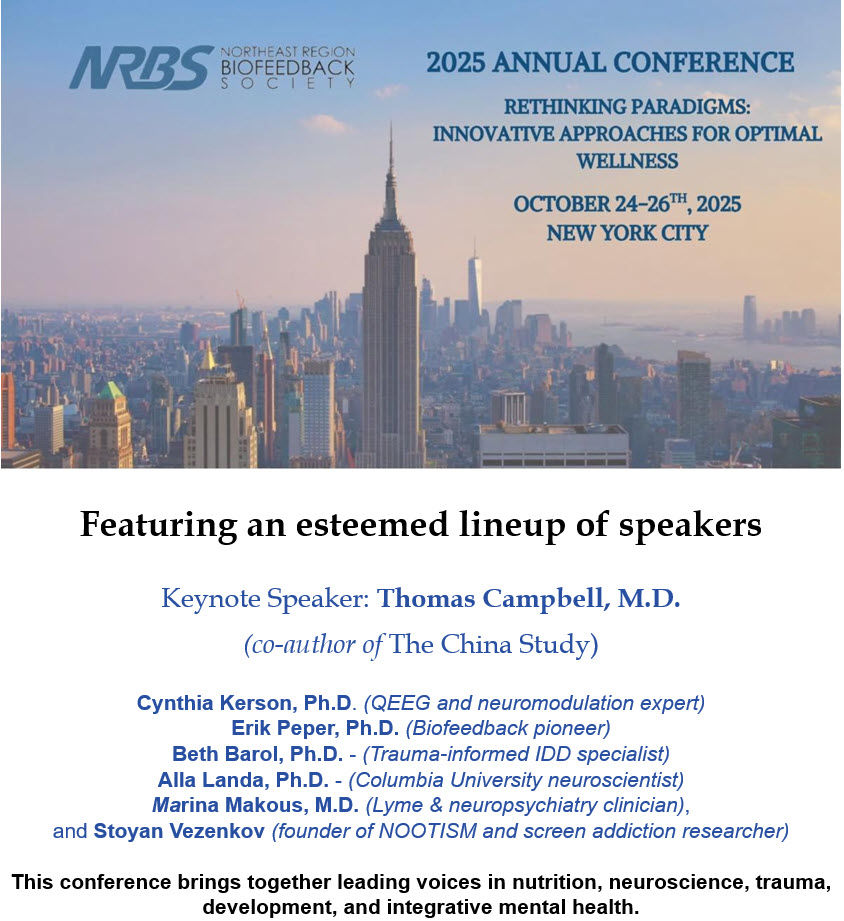
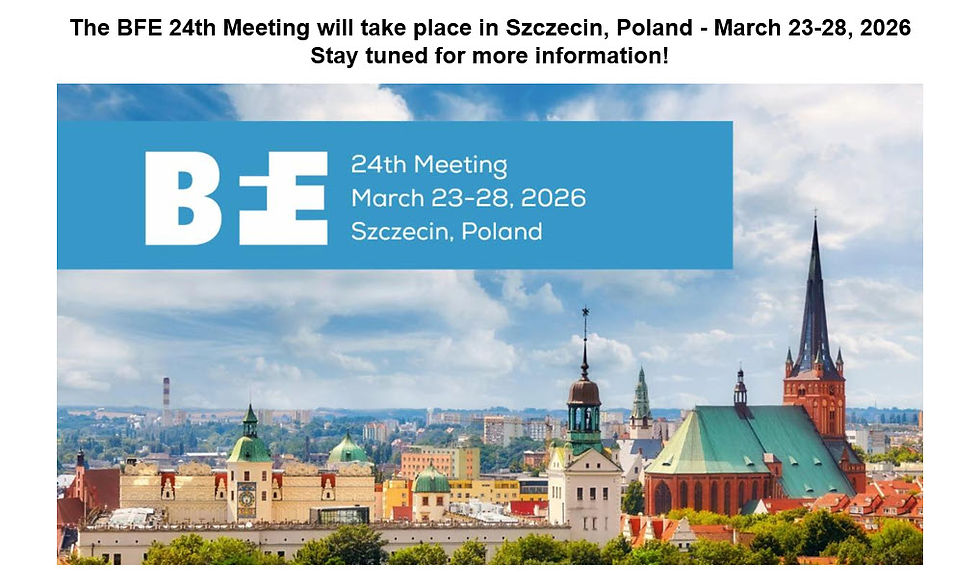
Comentários